Insights
KR Decarbonization Magazine
VOL.06 | Spring 2024
Biofuel as Marine Fuel
MOON Gunfeel, General Manager of KR Alternative Fuel Technology Research Team
![]() |
The Role of Biofuels in Alternative Marine Fuels
In the maritime industry, the application of various alternative fuels with high greenhouse gas reduction effects is anticipated to achieve goals of greenhouse gas reduction and carbon neutrality. Currently, carbon dioxide (CO₂), the primary greenhouse gas emitted from ships (Tank to Wake, TtW), is subject to greenhouse gas regulations. However, in the future, regulations are anticipated to encompass greenhouse gases with high Global Warming Potential (GWP), such as methane (CH₄) and nitrous oxide (N₂O), throughout the entire process, from raw material extraction to transportation, fuel production, and emissions (Well to Wake GHG emissions). Notable examples of such changes include the IMO’s LCA guidelines and the EU’s FuelEU maritime. In the future, under continually strengthened greenhouse gas regulations, fuels with recognized greenhouse gas reduction effects, particularly from a Well-to-Wake (WtW) perspective, should be used even in fossil-based alternative fuel propulsion systems.
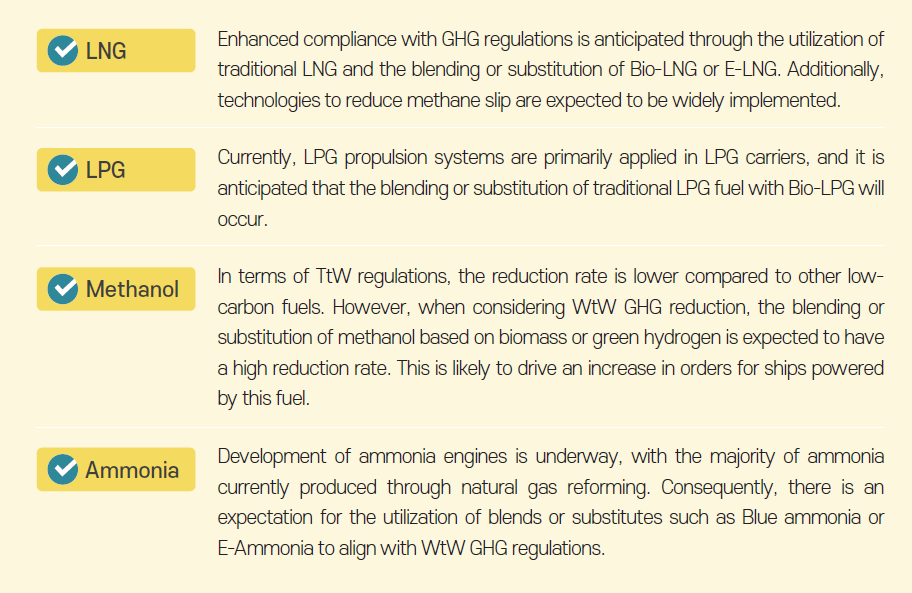
These alternative fuels each have their own advantages and disadvantages. It is anticipated that future shipping will consist of a mix of alternative fuel vessels, complementing each other. Biofuels are expected to play a vital role in meeting decarbonization objectives, especially in the initial phases of implementation towards achieving net zero emissions.
Biofuels are produced using raw materials such as biomass and can be blended or substituted with conventional fuels for use without any modifications to existing internal combustion engines and infrastructure. In other words, biofuels serve as ‘drop-in’ fuels that can be directly used in engine fuel systems without modification, prompting numerous shipping companies to conduct sea trials through the blending or substitution of biofuels. To implement biofuels, considerations must be made regarding quality, safety, cost-effectiveness, and environmental impacts.
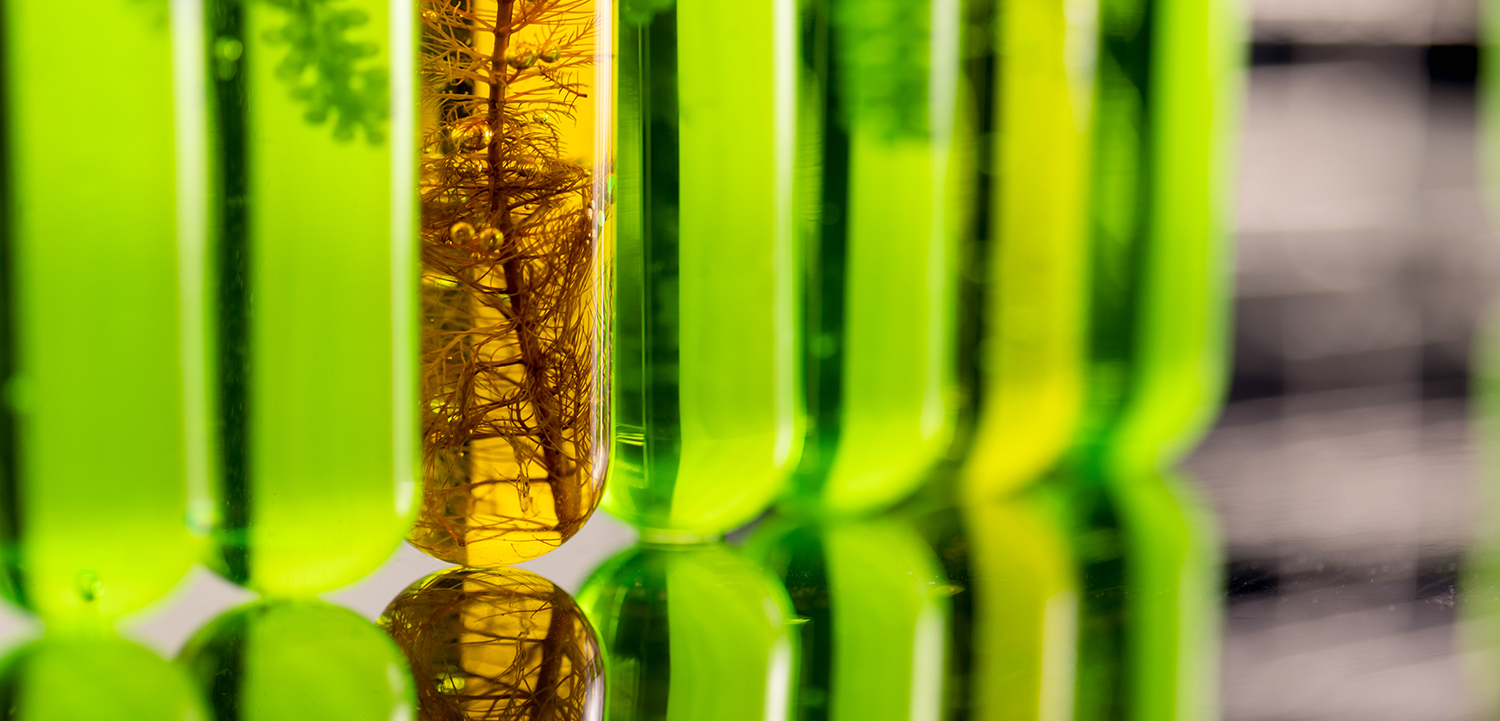
Types and Classification of Biofuels
Biofuels are environmentally friendly fuels produced from a variety of raw materials and manufacturing processes, including vegetable oils, animal fats, waste cooking oil, and wood waste. In this article the discussion is limited to biofuels that can be immediately applied in diesel engines or dual-fuel engines operating in diesel mode. The manufacturing processes and characteristics of each fuel are as follows:
1. FAME (Fatty Acid Methyl Esters)
FAME (Fatty Acid Methyl Ester), commonly known as biodiesel, is typically derived from raw materials such as vegetable oils, animal fats, and waste cooking oil. It is manufactured through an ester exchange reaction with methanol and the resulting fuel has an oxygen content of approximately 10%. FAME exhibits strong hydrophilicity, necessitating caution during long-term storage, and requires considerations regarding oxidation stability, low-temperature flow properties, and material compatibility.
2. HVO (Hydrotreated Vegetable Oils)
HVO (Hydrotreated Vegetable Oil) is produced using similar raw materials as FAME, such as vegetable oils or lignocellulosic biomass. This fuel is created through a hydrogenation process, similar to fossil fuel refining, where paraffinic hydrocarbons are formed through hydrogen treatment and decomposition processes. Due to the removal of oxygen-containing impurities during the manufacturing process, HVO fuel possesses properties similar to marine gas oil (MGO) and is suitable for long-term storage. However, its low viscosity requires verification of lubricity during use.
3. FP Bio-oil & HTL Bio-oil
Atmospheric pressure and oxygen-depleted, nitrogen-rich environments, typically at high temperatures (400~600℃), can create a process that thermally decomposes biomass to produce fuel. Fuel upgraded from this process is referred to as fast pyrolysis bio-oil. On the other hand, hydrothermal liquefaction bio-oil is derived from biomass that has been pulverized to an appropriate size, mixed with water, and subjected to hydrothermal liquefaction under high pressure and temperature conditions, resulting in liquid hydrothermal liquefaction bio-oil.
Both of these bio-oils have low technological maturity and require modifications to the fuel supply system for engine application. Therefore, this discussion primarily focuses on FAME and HVO, which are ‘drop-in’ fuels immediately applicable to engines.
Furthermore, biofuels can be categorized into generations based on their feedstock, which is closely linked to sustainability, environmental impact, and other factors. As a result, third-generation fuels, produced using microalgae, for example, have the lowest carbon intensity according to the WtW criteria, resulting in the greatest greenhouse gas reduction effect. However, they tend to have higher fuel purchase costs. On the other hand, first-generation fuels have lower purchase costs but relatively higher carbon intensity, resulting in lower greenhouse gas reduction effects. Therefore, it is essential to understand the tradeoff relationship between ease of compliance with GHG regulations and economic feasibility and adopt a strategic approach to the application of biofuels.
Comparison of Technological Maturity by Alternative Fuel
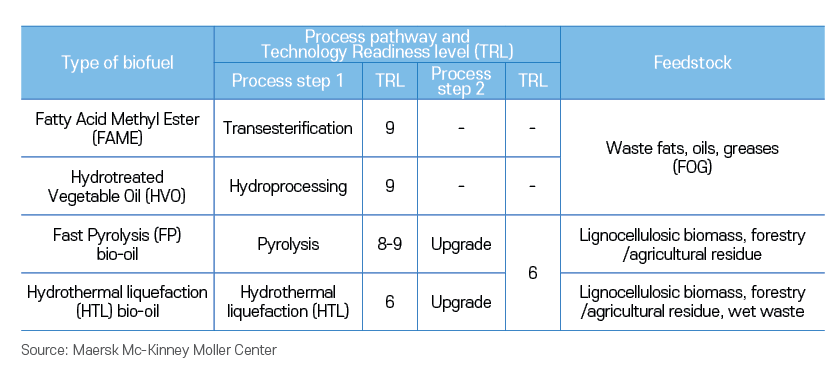
Comparison of Price and WtW Greenhouse Gas Intensity
According to Biofuel Generation Classification
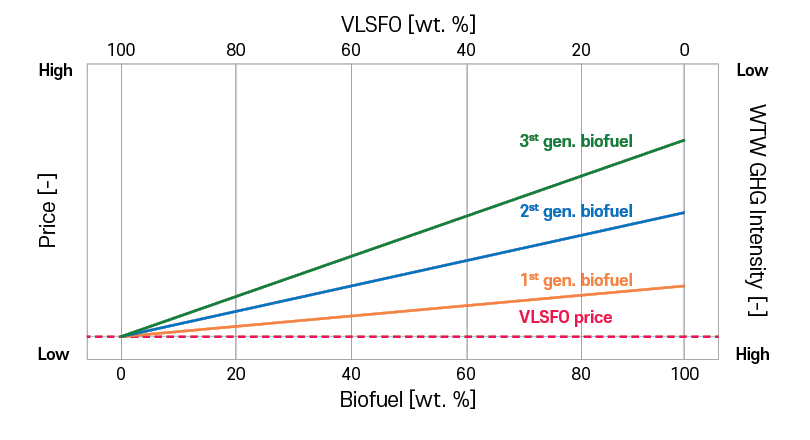
Biofuel Implementation Considerations
The international standards for biofuels currently used in ships are mainly limited to criteria for 7% v/v FAME and distillate fuel oil blends, with guidelines provided by the International Council on Combustion Engines (CIMAC). With the revision of ISO 8217, additional requirements for the blending of biofuels with conventional fuels are expected to be included. To accelerate net zero goals, there is a need for further international standard development to support the use of biofuels. Singapore has already taken the lead by adopting national standards allowing up to 50% v/v or m/m marine biofuel blends. While HVO fuel is not currently included in marine fuel standards, there are standards for paraffinic diesel fuel used on land.
Additionally, it is necessary to prepare for potential issues that may arise during engine operation. While maritime trials for biofuel use on ships are underway, they are primarily limited to short-term and small-scale biofuel or blend usage, making it difficult to establish standardized responses. Therefore, it is important to be aware of potential issues that may arise with long-term usage and to be prepared with measures to address them.
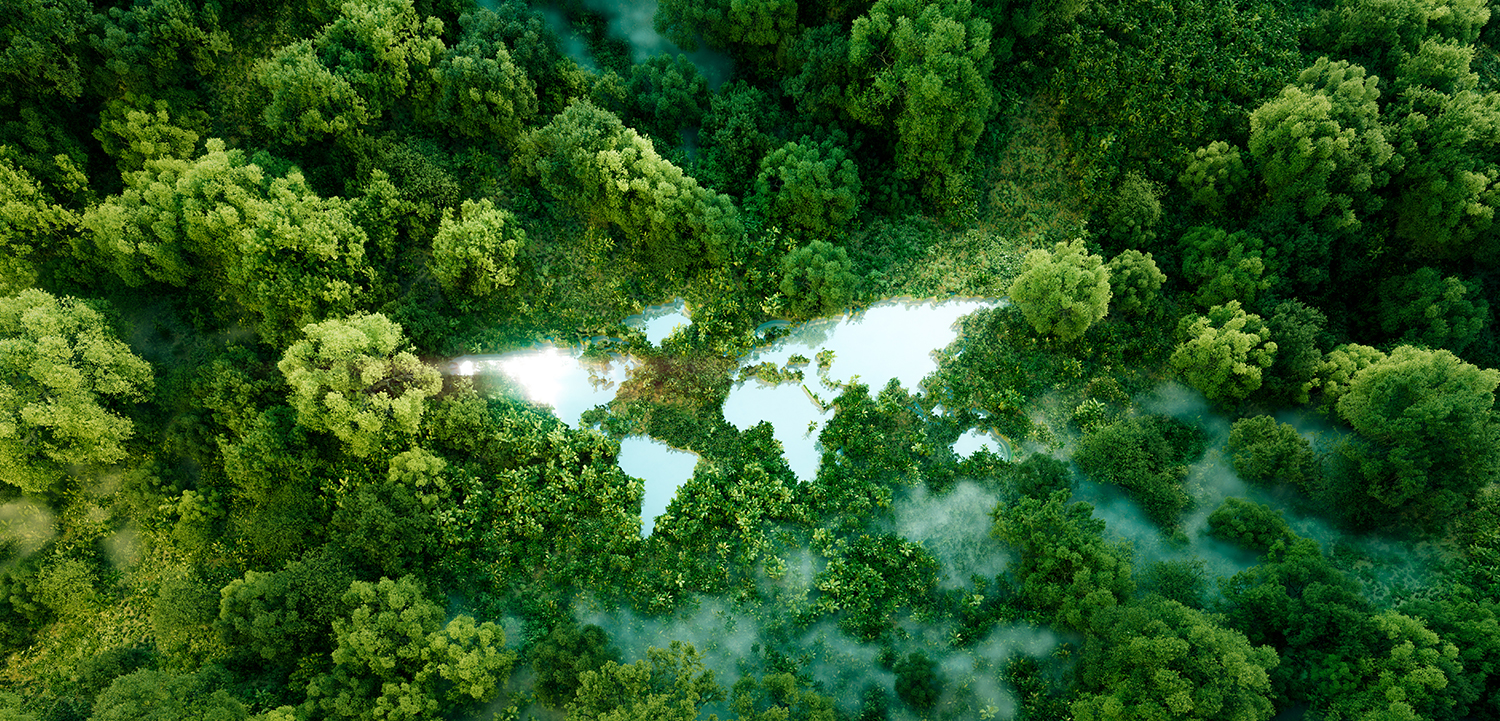
Firstly, microbial growth must be inhibited. FAME fuel, being highly hydrophilic, can suffer from fuel contamination by microbes during long-term storage. These microbes can cause sludge formation in fuel systems, as well as clogging of filters or pipelines. To prevent this, it is recommended to use small amounts of FAME fuel or blends as soon as possible. Temperature management of the fuel and tanks, as well as moisture removal, are necessary during long-term storage, and caution is required when using insecticides or other additives, as they may pose environmental and health risks. In contrast, HVO can be stored and handled in much the same way as conventional fuel.
Secondly, ensuring oxidation stability of biofuels is essential. Oxidation stability of fuel indicates its resistance to oxidation during storage and use. The unsaturated compounds in FAME fuel can increase its susceptibility to oxidation. Compounds formed as a result of oxidation can lead to clogging of filters, separators, and fuel injection devices, as well as corrosion of fuel systems. Considering the addition of antioxidants to enhance oxidation stability may be an option, although there are no known cases of such additives being added to biofuels supplied to ships. Additionally, since certain metal ions can promote oxidation, careful selection of materials for components is necessary.
Additionally, FAME exhibits reduced fluidity under low-temperature conditions, and the Wax Appearance Temperature (WAT) and Wax Disappearance Temperature (WDT) can vary depending on the feedstock or blend, necessitating proper temperature control. It is recommended to maintain the fuel temperature at least 10°C higher than the pour point, while cautioning against temperatures exceeding 40°C above the pour point to avoid the formation of gel-like polymers.
Lastly, certain metals such as copper, iron, lead, tin, and zinc can accelerate the oxidation process of FAME fuel, increasing the formation of precipitates. Furthermore, exposure to FAME fuel can cause swelling or deterioration of seals and gaskets, leading to equipment leaks or malfunctions. Therefore, it is essential to confirm the compatibility of components or materials with biofuels through equipment suppliers and engine manufacturers.
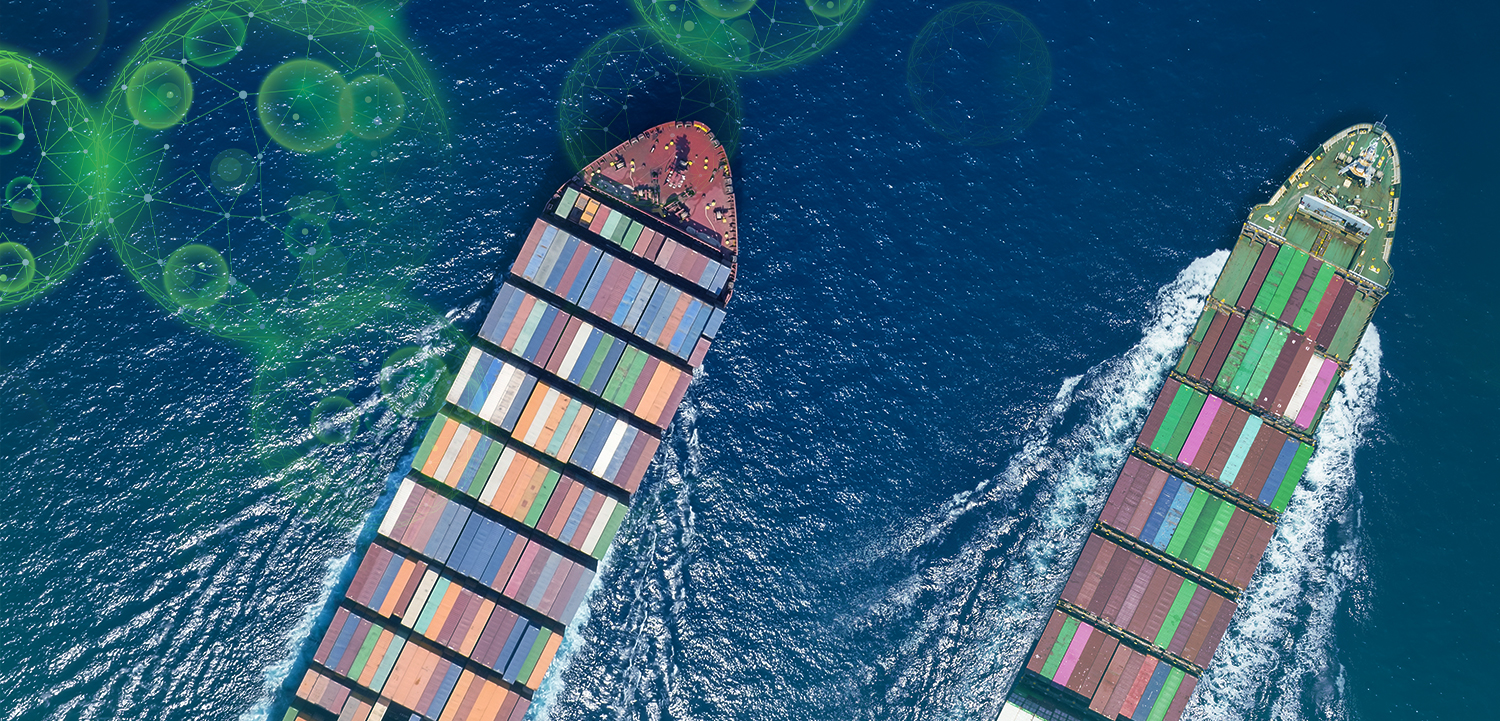
Production, Price, and Bunkering Infrastructure of Biofuels
The International Energy Agency (IEA) forecasts that by around 2050, the demand for bioenergy will be approximately 1.5 times higher than in 2022. It is especially expected that the supply of bioenergy produced from waste resources and woody biomass will increase in the future. In the shipping sector, the current usage of bioenergy, hydrogen, and hydrogen-based fuels, which is currently less than 1%, is anticipated to increase to approximately 15% by 2030 and up to 80% by 2050. As shown in Table 2, biofuels are expected to continue to grow not only in the shipping sector but also in the aviation sector, highlighting the significance of biofuels in achieving net zero.
Predicted Biofuel Usage Proportions by Transportation Sources
IEA Milestones for Biofuels | 2023 | 2030 | 2034 | 2050 |
---|---|---|---|---|
Biofuels Share in Road Sector | 5% | 11% | 12% | 3% |
Biofuels Share in Shipping | 0% | 8% | 13% | 19% |
Biofuels Share in Aviation | 0% | 10% | 22% | 33% |
Source : IEA
On the other hand, the use of bioenergy in various transportation sectors and almost all industrial sectors can trigger competition for sustainable biomass and biofuels, potentially leading to limitations in availability and price increases of biofuels in the shipping sector. While many institutions do not anticipate significant fluctuations in future biofuel prices, the expansion of biofuel usage in transportation and across industries is expected to result in price increases due to limitations in feedstock.
At present, the global infrastructure for bunkering biofuels is limited. However, with the increasing demand for sustainable fuels due to strengthened greenhouse gas regulations, the number of ports capable of bunkering is steadily growing. Nevertheless, regarding Ship-to-Ship (StS) biofuel bunkering, the standards specified for bunkering vessels in the IMO's IBC Code could pose initial barriers, prompting discussions on solutions, which are expected to commence from the 81st MEPC session of the IMO.
Regulatory Considerations for Biofuels
Major air pollutants emitted from ships include nitrogen oxides (NOx), sulfur oxides (SOx), particulate matter, among others, which are generated during the combustion process and can have environmental and human health impacts. Therefore, the IMO’s International Convention for the Prevention of Pollution from Ships (MARPOL) Annex VI regulates the emissions of these pollutants. Even when using biofuels for greenhouse gas reduction, air pollutants can still be emitted, so compliance with regulations must be verified based on the properties and combustion characteristics of the fuel.
The use of FAME, which includes oxygen in the fuel, is known to increase nitrogen oxide emissions, necessitating compliance with nitrogen oxide emission limits. To expedite compliance with greenhouse gas emission requirements, unified interpretations of the rules in Annex VI of MARPOL have been approved. Vessels utilizing biofuels blended at less than 30% can do so without undergoing a NOx verification process. If the mixture exceeds 30%, verification is required according to the NOx Technical Code 2008, unless confirmation is received from the engine manufacturer that the fuel can be used without changes to NOx-related components, settings, and operating values.
Biofuels, due to their low sulfur content, result in reduced sulfur oxide emissions when the biofuel blend ratio is increased, leading to a decrease in particulate matter emissions by reducing the formation of particles such as sulfuric acid or sulfate. FAME, containing oxygen, can improve combustion and reduce particulate matter emissions that may arise from incomplete combustion.
Regarding IMO DCS and CII greenhouse gas regulations, according to the provisional guidelines approved at MEPC 80, only biofuels that meet sustainability criteria and achieve a WtW GHG intensity reduction of over 65% (less than 33gCO2eq/MJ) compared to fossil fuels can calculate a CO₂ conversion factor based on a WtW GHG intensity. The CO₂ conversion factor for biofuel blends can be calculated through a weighted average. Fuels that do not meet these criteria will be subject to the CO₂ conversion factor of fossil fuels. It should also be noted that these provisional guidelines will be revoked once the LCA guidelines are finalized.
In Table 3, the improvement in Carbon Intensity Indicator (CII) resulting from the application of biofuels compared to conventional HFO fuel for a hypothetical vessel is presented. When applying biofuels or biofuel blends, it is assumed that they replace 50% of the usage of conventional fuel, while maintaining the same route and distance. Additionally, the WtW GHG intensity of biofuels is assumed to be 26.48 gCO2eq/MJ.
Comparison of Expected CII Ratings Based on Biofuel Application
Vessel Information (10,000 TEU Container) | Fuels* | 2023 | 2024 | 2025 | 2026 |
---|---|---|---|---|---|
Deadweight: 120,000 M/T | Case A | D | D | d | D |
Gross Tonnage: 114,200 M/T | Case B | C | C | C | D |
Distance Travelled: 70,000 Nautical Mile | Case C | B | C | C | C |
Fuel Consumption (HFO): 18,240 M/T | Case D | A | A | A | A |
* Case A : HFO 100% (Base), Case B : B30 (HFO 70% m/m, Biofuel 30% m/m)
Case C : B50 (HFO 50% m/m, Biofuel 50% m/m), Case D : Biofuel 100%
The results indicate that without taking any action and operating solely on conventional fuel (Case A) from 2023 onwards, the vessel maintains a D rating until 2026. However, when operating with a fuel blend containing 30% biofuel (Case B), it is projected to achieve a C rating by 2026. With a 50% biofuel blend (Case C), a B rating in 2023 and a C rating from 2024 onwards are expected. If the vessel switches entirely to biofuels (Case D), it is anticipated to maintain an A rating from 2023 to 2026. It’s important to note that these predictions are based on a hypothetical vessel and assumptions regarding the characteristics of biofuels (WtW intensity and lower heating value), so the actual improvement effects may vary for each vessel. Nevertheless, the use of biofuels or biofuel blends can be considered an effective solution for compliance with GHG regulations.
In relation to the EU’s greenhouse gas regulations, the ‘Fit for 55’ package has set a target to reduce greenhouse gases by 55% by 2030 compared to 1990 levels. Consequently, in the maritime sector, the EU Emission Trading System (ETS) has been implemented since January 2024, requiring ships entering and leaving EU ports to purchase emission allowances through emissions accounting. Additionally, the FuelEU Maritime regulation is scheduled to be implemented from 2025, mandating the use of environmentally friendly fuels on ships, with penalties for non-compliance. Biofuels, recognized as sustainable fuels under EU renewable energy guidelines, are expected to see expanded use as they can be assigned as zero coefficient.
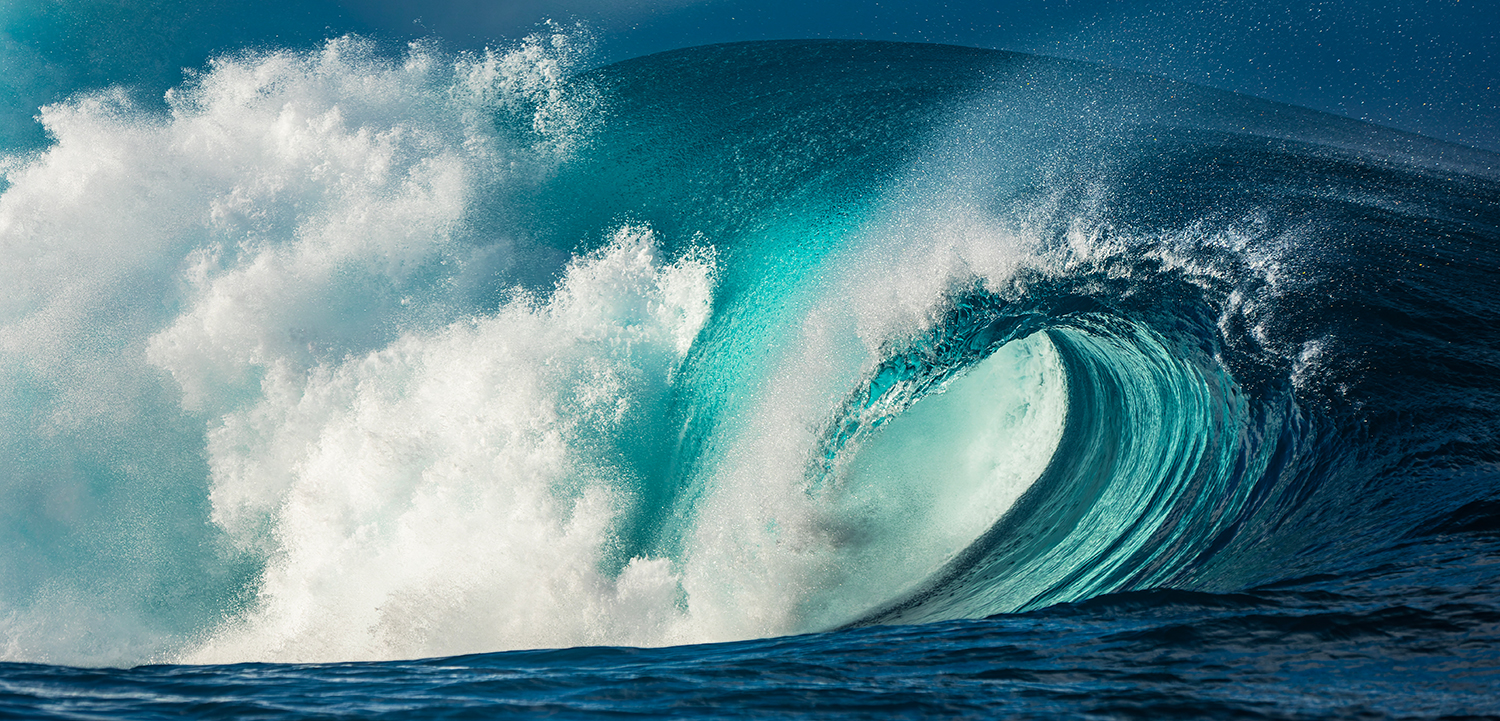
Summary and Recommendations
Derived from biomass, biofuels share similarities with fossil fuels, and some can be readily utilized in existing internal combustion engines. This characteristic has drawn attention from shipping companies and shippers, primarily because of their favorable compliance with greenhouse gas regulations. Biofuels generally have higher technological maturity and fewer issues to resolve compared to other zero-carbon fuels. Furthermore, concerning regulatory compliance, the utilization of biofuels can enhance CII grade ratings, as biofuels with lower WtW carbon intensities are anticipated to offer significant regulatory advantages.
However, despite the sustainability and green-house gas reduction potential of biofuels, there are several technical challenges, including the absence of fuel quality standards for marine biofuels. Additionally, the availability and price of biofuels may be unstable due to resource limitations and competition with other industries. Therefore, the shipping industry needs to develop medium to long-term strategies considering both the technological and economic aspects of biofuels.
KR recognizes the importance of biofuels in responding to greenhouse gas regulations and has been actively publishing technical documents, regulatory newsletters, and conducting research activities in this regard. Recently, KR published a technical document titled ‘Biofuel as Marine Fuel’ to facilitate understanding of biofuel use in relevant industries. In collaboration with the industry, an MOU has been signed with domestic shipping companies, engine manufacturers, and fuel suppliers. Maritime trials for bio-blended fuels were successfully conducted with a 13,000 TEU container vessel in March 2021. Presently, we are collaborating with both domestic and global shipping companies on research initiatives to promote biofuel usage and formulate safety guidelines. Furthermore, technical services are provided for measuring and analyzing engine performance as well as air pollutants (including GHG) using biofuel or biofuel blends on the low-speed engine (7.4 MW) test bench at our Greenship Test and Certification Center (TCC).
Ultimately, KR is committed to collaborating continuously with relevant industries until the activation and safety of biofuel use are ensured. This commitment underscores our crucial role in the early implementation of greenhouse gas reduction efforts towards achieving net zero emissions.
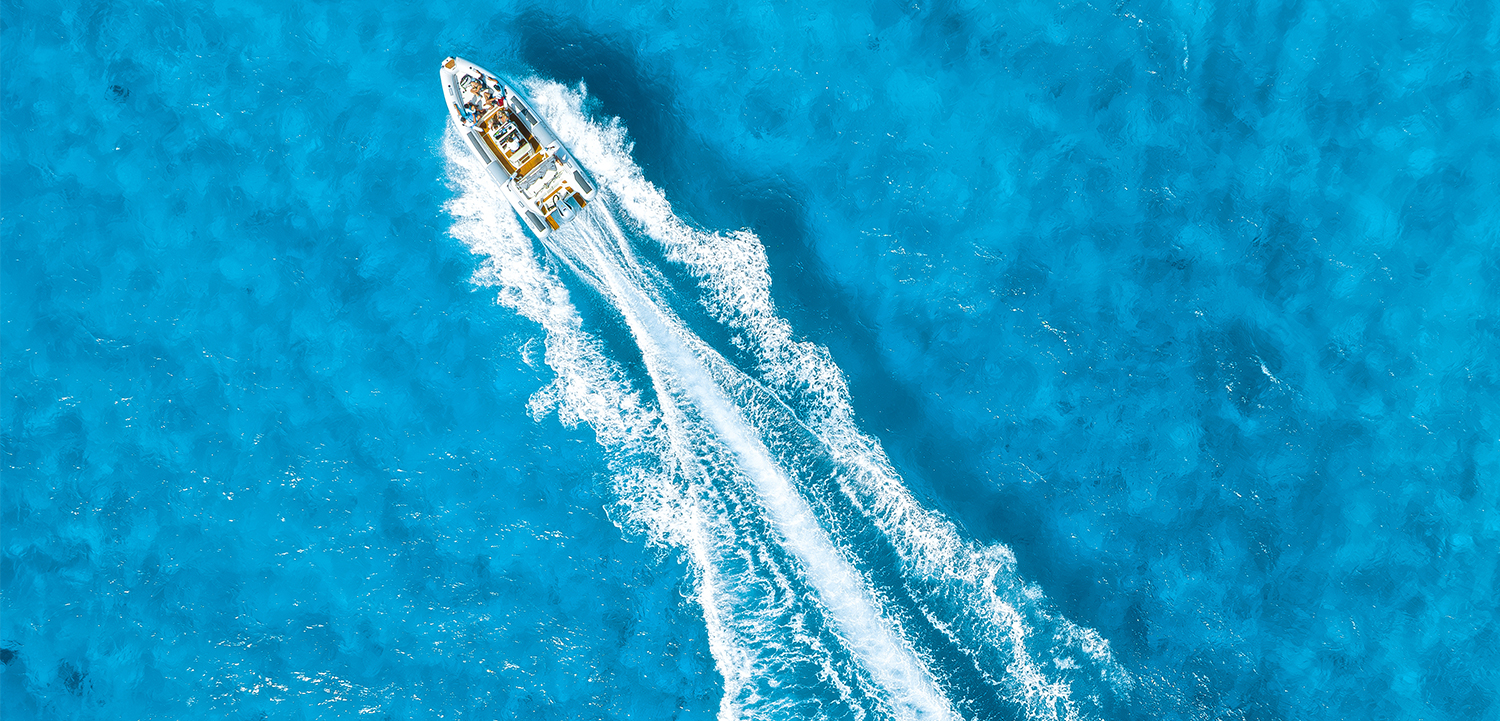
Market and Key Technologies for the conversion to Eco-Friendly Methanol Fueled Container ship
PARK Seungmin, Senior Surveyor of KR Dry Cargo Ship Team
![]() |
Efforts for Carbon-Neutral and Methanol Fuel
Amidst the increase in greenhouse gas emissions leading to global warming and severe issues like climate change, the International Maritime Organization (IMO) has adopted the ‘2050 Carbon net-zero target’ at 80th MEPC session. As many countries and companies intensify their efforts to achieve carbon-neutral, methanol, along with LNG, is primarily being chosen as an eco-friendly ship fuel at the current time. Especially, according to Clarkson data as of February 5th this year, out of the total 207 newly contracted container ships worldwide last year, eco-friendly fuel ships accounted for 129 vessels, about 62%, among which, methanol fuel propulsion ships contracts were 86 vessels (41.5% of the total), double the number of LNG fuel propulsion ships at 43 vessels (21% of the total). The reason methanol is gaining attention is that it can significantly reduce the emission of pollutants such as sulfur oxides (SOx) and nitrogen oxides (NOx) compared to conventional fuels, and it is possible to supply green methanol, which is bio-methanol or e-methanol, with practically zero (carbon-neutral) greenhouse gas emissions.
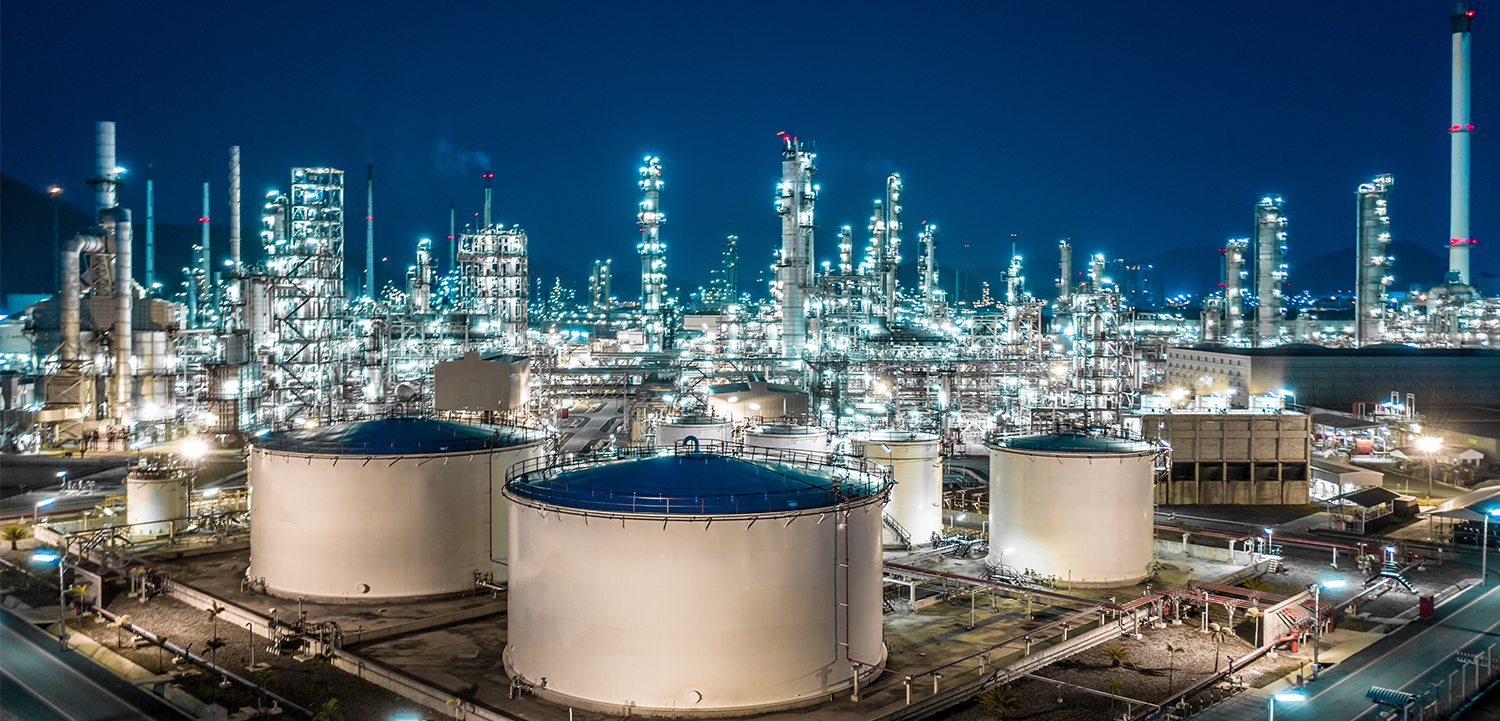
Conversion to Methanol Fueled Container Ships
From the shipping company’s perspective, converting existing container ships to eco-friendly fuel (Dual Fuel) propulsion involves considering the ease of conversion, scope and cost of conversion, cargo loss, bunkering, etc. Importantly, unlike other eco-friendly fuels, methanol is in a liquid state at room temperature, making it easy to store on ships without the need for cryogenic independent tanks or membrane-type fuel tanks required by LNG, allowing methanol fuel tanks to be integrated into the ship’s structure using general structural steel. For this reason, converting operational ships to eco-friendly fuel ships is relatively easier in terms of ship structure and layout when applying methanol fuel. For the conversion to methanol dual-fuel ships, main engines, auxiliary engines, generators, fuel supply systems, and methanol fuel tanks need to be supplied. Depending on the size of the ship, the engines applied, and the capacity of the methanol tanks, about 20% of the cost compared to a new building container ship is required. Additionally, an optimized conversion plan and process work must accompany the effort to retrofit an operating ship.
During conversion, part of the existing container cargo hold (1-2 bays) should be converted into fuel tanks, which can result in up to a 4% loss of existing container cargo for ships over 10K TEU(twenty equivalent unit) class. The stable supply of methanol fuel must also be considered, with currently about 120 million tons produced annually at around 90 production sites worldwide. According to the 『Methanol as a Marin Fuel』 published by KR, the future methanol production market is expected to grow, with current production growth rates indicating that production could increase from 120 million tons in 2025 to 500 million tons by 2050.
Conversion Market for Methanol Fueled Container Ship
Currently, major container shipping companies like Maersk and CMA CGM are increasing the proportion of methanol fuel propulsion container ships through not only new builds but also conversions of part of their existing fleets. In November last year, Maersk contracted with China’s Zhoushan Xinya Shipyard for the first project to convert an operational container ship into a methanol dual-fuel propulsion ship. The methanol dual-fuel engine for the conversion is from Germany’s Man-Energy Solution, and the targeted ships for conversion are known to be 11 vessels, with the first ship expected to undergo about 3 months of conversion work starting from June this year. CMA CGM has signed a project contract with China’s CSSC Group’s Qingdao Beihai Shipbuilding for the conversion of 8 operational container ships of 9,200 TEU to methanol duel fuel propulsion. In the future, major container shipping companies including HMM, HAPAG-LLOYD, and SEASPAN are also pushing for methanol duel-fuel propulsion ship conversions, with currently about 70 large operational container ships planned to be converted to methanol dual-fuel propulsion.
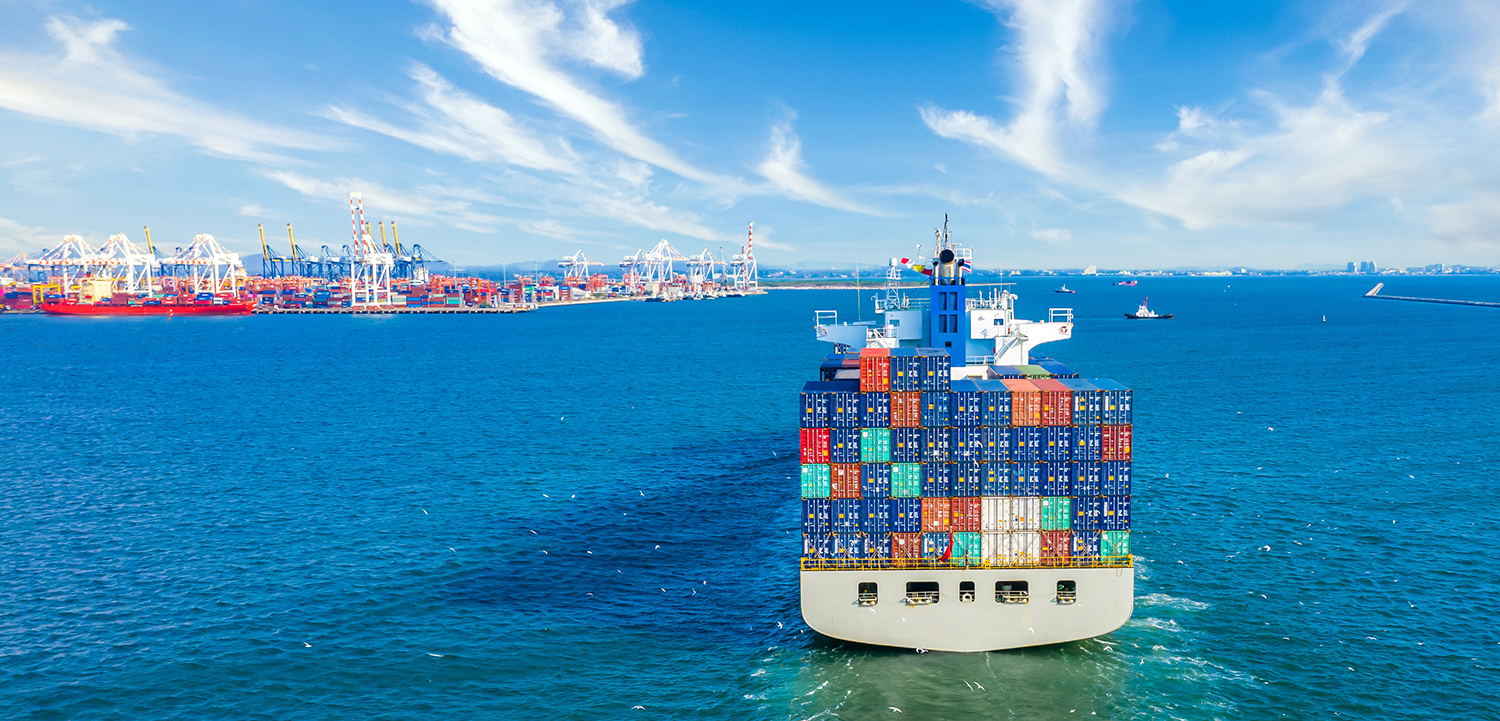
Joint Development for the Conversion of a 16,000TEU Large Container Ship
In Korea, ‘HD Hyundai Marine Solution (HD HMS)’, a comprehensive marine industry solution company of HD Hyundai, has started a business in the field of eco-friendly decarbonized methanol dual-fuel propulsion ship conversion. HD HMS carried out a joint development project (JDP) for methanol fuel propulsion ship conversion with KR, HD Hyundai Heavy Industries (HD HHI), and HD Hyundai Engineering & Technology (HD Hyundai E&T), targeting HMM’s largest 16,000 TEU container ship in operation, and obtained basic certification (AIP, Approval In Principle) from KR at the end of 2023.
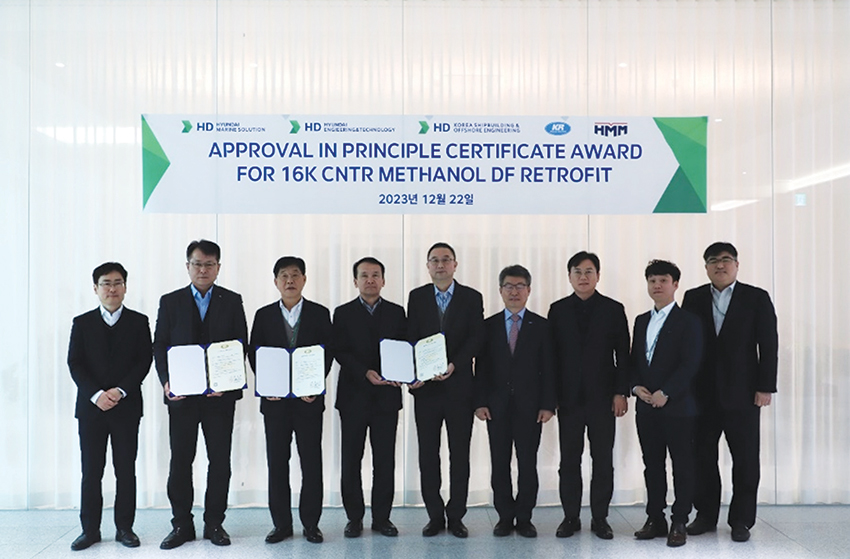
Technologies for Methanol Fuel Propulsion Ship Conversion
HD Hyundai Marine Solution performed the basic design for the system configuration for the conversion of large container ships to methanol fuel propulsion ships, and HD Hyundai ENT was responsible for 3D modeling and detailed design. Additionally, a newly developed ‘Low Flashpoint Fuel Supply System (LFSS)’ by HD Hyundai Heavy Industries was applied, and the design was based on MAN’s main engine and HD Hyundai Heavy Industries’ own developed methanol dual-fuel generator engine, the HIMSEN engine. The methanol dual-fuel engine, generator, low flashpoint fuel system, tanks, and cofferdams were designed and arranged in compliance with the IMO’s MSC.1/Circ.1621 Interim Guideline.
The existing container cargo hold located in front of the engine room’s bulkhead has been modified to load methanol fuel, enabling it to operate on the Europe-Asia one-way route. The material for the methanol fuel tank is generally carbon steel applied to the ship’s hull, but considering the corrosiveness of methanol, a special zinc silicate coating was applied. The methanol fuel tank was designed as one large block to minimize the conversion time and cost of existing ships and facilitate the conversion work. Additionally, the tank’s support structures were placed at key locations to efficiently connect and weld to the existing ship structure.
The fuel tank was designed to be suitable for the harsh environmental loads and internal loads required by KR’s rules for classification of Steel ships. A direct strength assessment was conducted for various fuel oil tank/container loading scenarios to evaluate yield strength, buckling strength, etc. Especially, structural stress concentrations around the main support structures connecting the hull and tank were identified through detailed fine mesh analysis, and appropriately reinforced.
Design for methanol fuel propulsion ship conversion
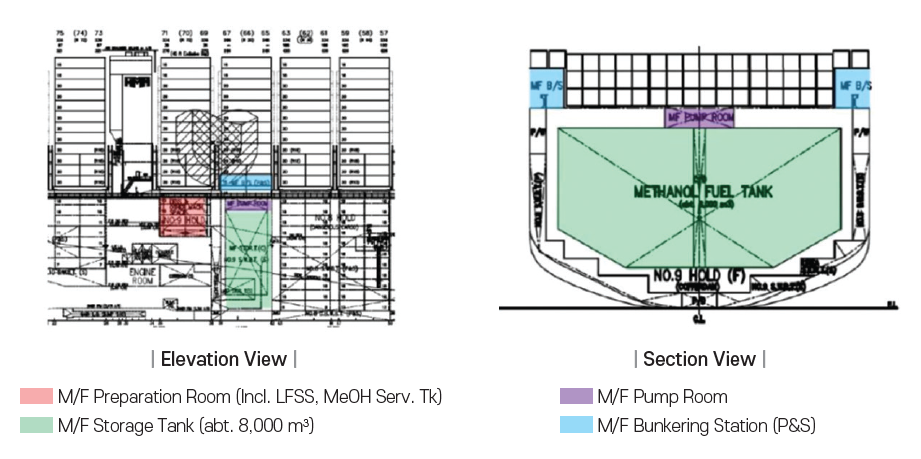
Structural analysis of methanol fuel propulsion ship conversion
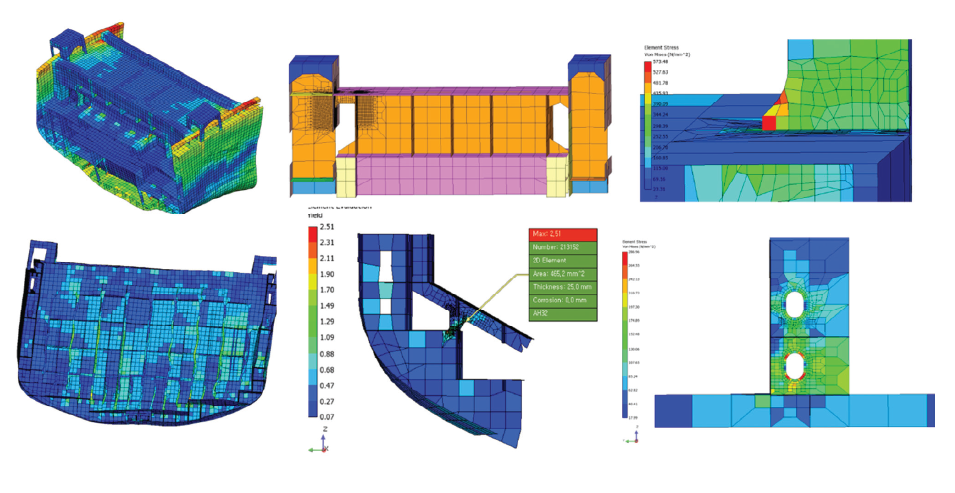
KR's roles
KR has actively supported HD Hyundai Marine Solution to secure methanol duel fuel propulsion conversion technology through this joint project, as well as maintaining an active technical support and cooperation relationship with major shipyards, shipping companies, and makers in conducting joint research and new builds of methanol dual-fuel propulsion large container ships, including engine, structure, equipment layout, convention requirements, and risk assessment (HAZID & HAZOP). KR plans to expand its role as a trusted partner to help our customers swiftly respond to the decarbonization era and explore new areas through such close cooperation.
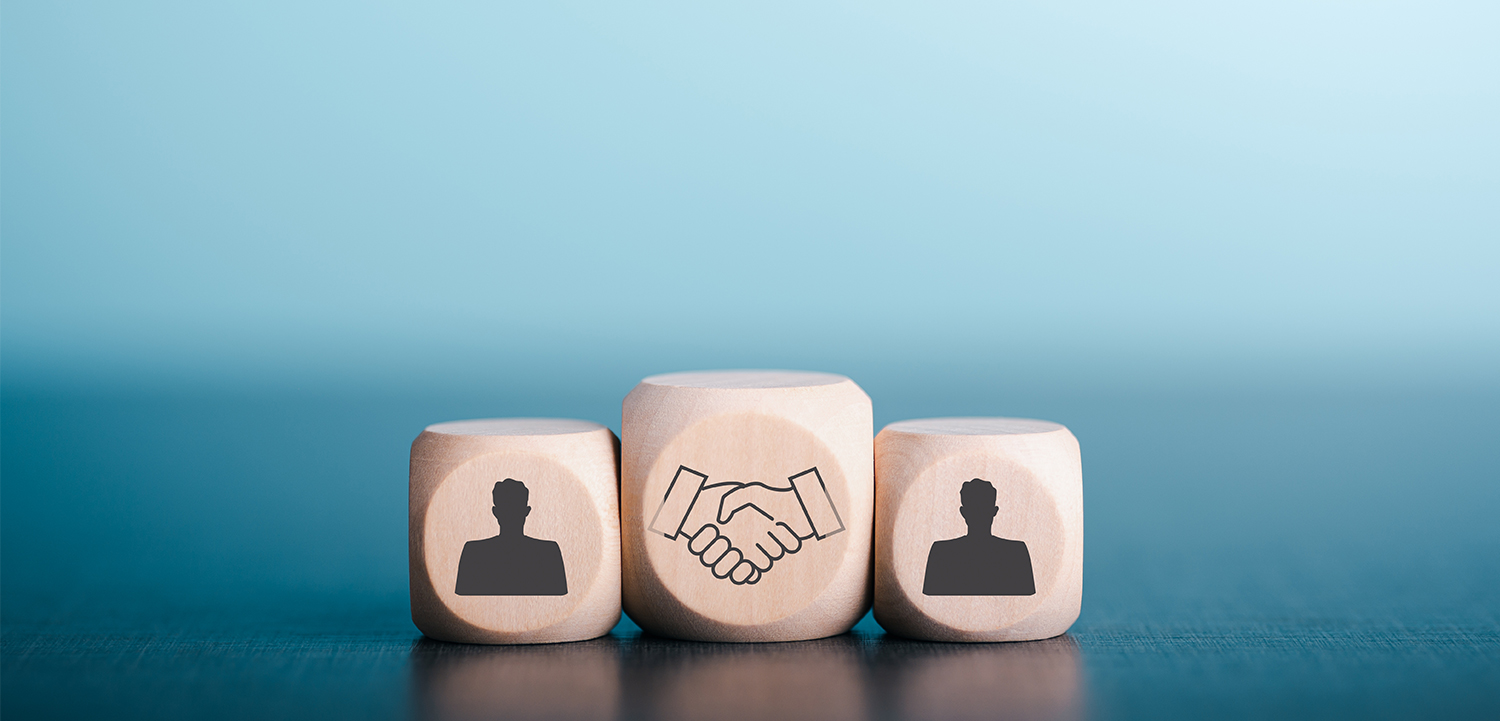